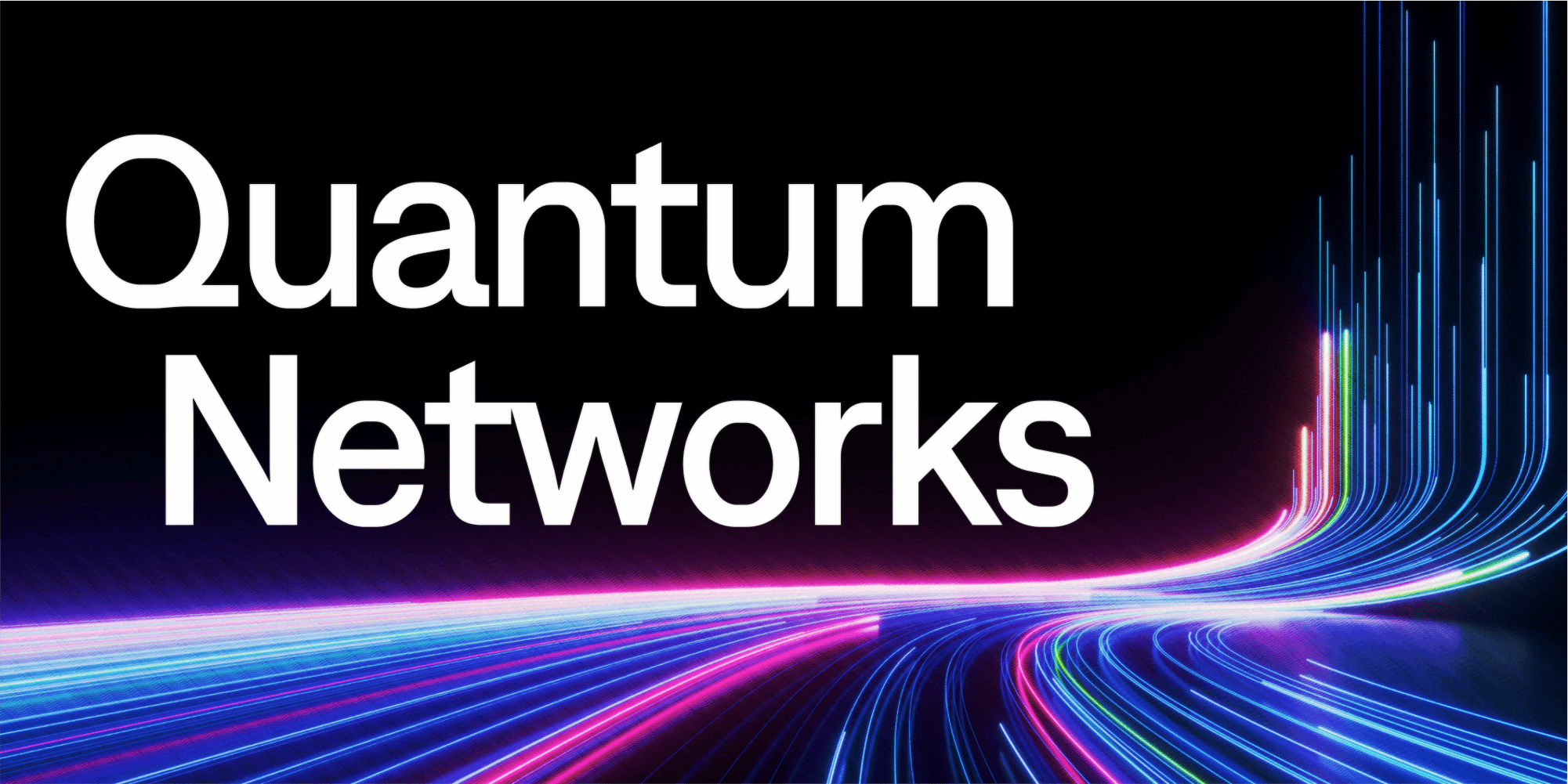
Quantum networks are a rapidly developing area of quantum technology that could revolutionize secure communication. By harnessing the principles of quantum mechanics, such as superposition and entanglement, quantum networks aim to transmit information in a way that is fundamentally different from classical networks. This gives quantum networks advantages over classical networks, including security based on the laws of quantum mechanics, enhanced privacy and protection against eavesdropping, and the potential for exponential speedup in certain computational tasks.
Quantum networks have the potential to enable various applications, such as quantum key distribution (QKD) for unbreakable encryption, distributed quantum computing, and enhanced sensing capabilities. However, building large-scale quantum networks poses significant challenges, including the generation, transmission, and detection of quantum states, as well as the development of quantum repeaters to extend the range of quantum communication.
Despite these challenges, researchers around the world are making progress in developing quantum network technologies. From small-scale demonstrations of QKD to the development of satellite-based quantum communication, the field is advancing rapidly. As quantum networks continue to evolve, they may one day form the backbone of a global quantum internet, enabling secure communication and powerful distributed computing applications.
What are Quantum Networks?
Quantum networks facilitate the transmission of quantum data or qubits, mainly stored in individual photons, between physically separated nodes. In a quantum network, information is encoded in the quantum states of particles, typically photons. These photons can be entangled, meaning their quantum states are correlated even when separated by large distances.
One key advantage of quantum networks over classical networks is that faster transmission of encoded information can be achieved with the aid of two core quantum phenomena: superposition and entanglement. Superposition allows a quantum system to exist in multiple states simultaneously, while entanglement enables instantaneous correlations between particles, regardless of the distance between them. These quantum properties enable the development of novel communication protocols and algorithms that can outperform their classical counterparts in terms of speed and efficiency.
Compared to classical networks, quantum networks are more secure due to these quantum elements, which makes hacking more difficult because of the fragility of quantum states and how they are destroyed on measurement, and the no-cloning theorem – quantum states cannot be copied. The no-cloning theorem, a fundamental principle of quantum mechanics, states that it is impossible to create an identical copy of an unknown quantum state. This property allows for the creation of secure communication channels, as any attempt to intercept or measure the photons would disrupt their entanglement and alert the communicating parties.
Furthermore, quantum key distribution (QKD) protocols, such as BB84 and E91, leverage the principles of quantum mechanics to establish secure keys between communicating parties. These protocols ensure that any attempt to eavesdrop on the communication would be detected, as measuring the quantum states of the photons would inevitably disturb them, revealing the presence of an intruder.
Components of A Quantum Network
Like classical networks, quantum networks are made up of different components, all of which are responsible for the quantum network’s functionality:
- Quantum Nodes
These are quantum processors for generating, manipulating, and measuring qubits. Quantum network topologies deploy spatially detached or interconnected nodes in a quantum network. These nodes are concerned with exchanging qubits and providing the path for transmitting and receiving quantum information. Also, quantum nodes can serve as short-term memories for storing quantum states.
- Quantum Channels
Quantum channels are the physical media for transmitting quantum states, such as optical fibers and free space while maintaining quantum coherence and mitigating noise. Quantum networks can be propagated over telecommunication optical fibers similar to those used for existing classical networks. In 2020, a team of researchers affiliated with several Chinese institutions succeeded in sending entangled quantum memories over 50 kilometers (31 miles) of standard fiber optic cables.
- Quantum Repeaters
Quantum repeaters are devices for extending the range of quantum communication. They create entangled states between remote quantum nodes by combining a series of basal entanglements on individual links. Quantum repeaters rely on a technique known as entanglement swapping. This technique involves the transfer of entanglement to a pair of qubits (photons especially) that were produced separately and without any prior interaction whatsoever. Quantum repeaters also rely on yet another technique known as entanglement purification. This allows for the creation of near-maximally entangled qubits from a large number of random weakly entangled qubits and provides additional protection against errors.
Quantum Network Implementations
While quantum networks are still under development, there have been demonstrations and live implementations of quantum networks across the world:
- The DARPA Quantum Network, which ran from 2003 to 2007, was the world’s first QKD network. It was built on 10 optical nodes across Boston and Cambridge, Massachusetts. The first phase of the network became operational in October 2003 in BBN's laboratories; later, in June 2004, the network was extended to two nearby universities, using dark fiber running under the streets of Boston and Cambridge. While it created the first network of interconnected nodes, DARPA’s Quantum Network lacked the technology for large-scale quantum networks, such as quantum repeaters. However, this demonstrated a huge step towards the development of future quantum networks.
- In 2010, NICT, Japan’s National Institute of Information and Communication Technology, inaugurated a metropolitan QKD network in Tokyo, Japan. It was constructed on an open test-bed network known as “Japan Giga Bit Network 2 plus" or “JGN2plus,” with four access points situated in Koganei, Otemachi, Hakusan, and Hongo. The project brought together several Japanese and overseas companies and academic institutions to build six optical network links of the QKD network: NEC, NTT, Mitsubishi Electric, Austrian Institute of Technology (AIT), Toshiba Research Europe, and ID Quantique.
- In 2017, China launched the world’s first longest trunk line for quantum telecommunications. The Jing-Hu or Beijing-Shanghai trunk line spans 2,000 km and uses integrated fiber-based QKD network links to connect Beijing, Jinan, Hefei, and Shanghai. The line is connected to the world’s first quantum satellite, which China launched in 2016. The satellite is nicknamed “Micius,” after a 5th-century B.C. Chinese philosopher and scientist who has been recognized as the first person to conduct optical experiments.
- Quantum Internet Alliance (QIA) is a consortium of 40 multidisciplinary academic, research, and technology partners founded in Europe in 2017. The Alliance is working collaboratively to build what they term “the world’s first full-stack prototype quantum internet network.” The aim of this flagship initiative is to establish a quantum network infrastructure that is capable of steering Europe’s quantum internet ecosystem to new heights. QIA looks to achieve entanglement and teleportation that spans three to four remote quantum network nodes, making the transition from simple point-to-point connections to the first multi-node networks possible.
- In 2020, the United States, through the Department of Energy (DOE), announced a report outlining its blueprint for the quantum internet. This report lays out a development pathway for the National Quantum Initiative Act, which was signed into law by President Donald Trump in December 2018. The blueprint specifies plans to build the nation’s first large-scale quantum networks, which will position the U. S. at the forefront of the global quantum race and usher in a new era of communication and connectivity.
Future Outlook for the Realization of Quantum Networks
Though quantum networks still require research and expansion, the government, military, and financial sectors already use quantum telecommunication protocols.
Even though quantum networks are being implemented across the world, the IEEE Standards Association, the international body tasked with developing and maintaining quantum standards, including quantum network protocols, has not yet released standards in quantum telecommunication. IEEE P1913 is the standard for the Software-Defined Quantum Communication protocol, which is the framework for dynamically designing, creating, operating, modifying, or removing quantum network protocols and applications. This standard also aims to facilitate the interoperability and compatibility between different quantum network components, which can lead to more cooperation between regions and countries to create a global quantum internet.
However, even widespread coverage for regional and global quantum communication still poses challenges and presents areas for further research:
- Development of high-fidelity quantum processors and memories.
- Improvement of quantum error correction and fault-tolerant techniques.
- Exploration of new materials and platforms for quantum network components.
The expected timeline for the widespread adoption of quantum networks is by 2030. As research into quantum networks progresses, we inch closer to realizing a quantum internet – a global network of interconnected quantum devices and computers. This quantum internet could usher in a new era of secure communication, advanced scientific collaboration, and unprecedented computational capabilities.